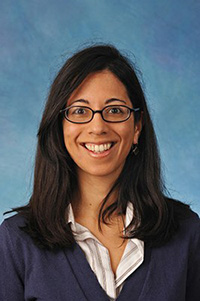
Maya Styner, MD
Dr. Maya Styner’s lab is interested in how exercise benefits health, specifically bone marrow health. Our bones are not stagnant, rock-like materials – they change throughout life. The marrow within bones contains various kinds of cells, including fat cells. The proportions of these cells can decrease or increase over time. Bone marrow fat is known to be increased in clinical conditions of bone fragility, such as osteoporosis. To measure the amount of fat in bone, in mouse models for instance, has required microscopic histomorphology on small bone slices; using such a tedious method has led to uncertain understanding of the contribution of fat cells to bone health because bone slices don’t provide a clear picture of the entire amount of fat in bone. Dr. Styner, along with her research mentor Dr. Janet Rubin and collaborators in medical image analysis at UNC, developed a quantitative means for reproducibly quantifying bone fat by microCT. This quantification method allowed her team to rigorously assess bone fat: for the first time they showed conclusively that marrow fat increases with high fat feeding. Further, female mice like to run – an average of 11 km nightly – and with this, bone formation increases. With the increase in bone formation, and perhaps due to energy expenditure, bone marrow fat is significantly repressed by exercise in running mice.
Dr. Styner is currently conducting experiments in obese and in lipodystrophic mice to further understand the relationship between exercise, bone fat and bone formation. Dr. Styner’s background in Endocrinology and skeletal biology, combined with an interest in exercise physiology, make this an exciting venue for research into diet and exercise, which may be the most challenging issues facing the modern world.
Dr. Styner’s contributions can be viewed on ResearchGate and on LinkedIn.
Listen to Dr. Styner’s interview with Dr. Falk on her research in bone marrow fat.
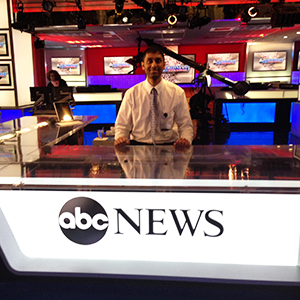
M. Sue Kirkman, MD
The Diabetes Care Center’s Clinical Trials Unit has conducted more than 200 studies over the last 20 years. The diabetes center’s success is based on the recognized need for new ways to prevent, detect and treat diabetes and its complications. This reality inspires our patients, coordinators, research assistants, managers and faculty.
Founded in 1994 by John Buse, MD, PhD, the Clinical Trials Unit has been directed since 2014 by M. Sue Kirkman, MD, who leads the team of approximately 20 faculty and staff currently conducting about 15 investigator-initiated and contracted research protocols involving hundreds of research volunteers and funded by the NIH, PCORI and industry. Dr. Buse is the site PI of multiple clinical trials and a member of the Executive Committee of the NIH-funded multi-site Glycemia Reduction Approaches in Diabetes: A Comparative Effectiveness Study (GRADE) trial. Dr. Kirkman is the site P.I. of the GRADE trial, a long-term real-world study to determine the comparative effectiveness of common therapies for type 2 diabetes, and also the site P.I. for several other trials. Laura Young, MD, PhD is co-PI of a PCORI-funded project on glucose monitoring and is interested in the interface of treatments, technologies, training and patient behaviors to improve diabetes outcomes. Jean Dostou, MD leads trials in type 1 diabetes prevention. Beth Harris, MD, Kate Bergamo, BSN, RN, MS, FNP-C and April Goley, FNP-C round out the team of investigators.
The unit has provided leadership or participated in many of the key diabetes studies and developments that define modern diabetes care, from novel drug approaches such as the combination of GLP-1 receptor agonists and basal insulin to technologies such as continuous glucose monitoring, insulin pump therapy, and the “bionic pancreas.” A listing of team members, currently recruiting studies, and links to our patient registry are available at www.uncdiabetes.org.
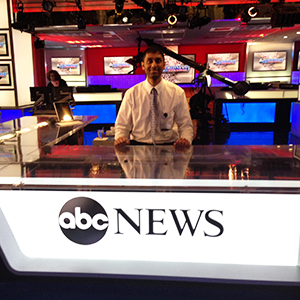
Eric Klett, MD
Dr. Eric Klett is interested in the role of intra-pancreatic beta-cell fatty acid metabolism and glucose-stimulated insulin secretion (GSIS). Type 2 Diabetes mellitus (T2DM), which accounts for 90 to 95% of diabetes worldwide, is characterized by resistance of peripheral tissues to the actions of insulin, together with a relative deficiency of insulin secretion. Both insulin resistance and pancreatic beta-cell dysfunction are present early in the development of T2DM, but hyperglycemia (i.e. diabetes mellitus) does not develop without beta-cell dysfunction. Insulin secretion is a complex process initiated by nutrient secretagogues, including glucose and fatty acids. GSIS is augmented by saturated long-chain fatty acids, but is impaired by ω-6 polyunsaturated fatty acids (PUFA). Glucose and fatty acids are essential to GSIS, though the exact molecular mechanism by which specific fatty acids alter GSIS remains unclear.
Dr. Klett’s lab has examined the role of one of the rate-limiting enzymes in glycerolipid synthesis on beta-cell function, acyl-CoA synthetase (ACSL). Specifically, exposing beta-cells to ω-6 PUFAs (arachidonate or linoleate) not only impairs GSIS, but also reduces ACSL isoform-4 (ACSL4) mRNA and protein expression. Further, reducing ACSL4 specific activity decreases GSIS. The reduction in insulin secretion is due to the accumulation of unesterified epoxyeicosatrienoic acids (EETs). Additionally, they found that EETs can serve as a substrate for ACSL4, indicating that this enzyme may be essential in the metabolism of not only EETs but also, perhaps, all eicosanoids. The hypothesis that ACSL4 plays a key role in eicosanoid metabolism has significant implications in all disease processes that involve eicosanoid metabolism, including diabetes, cardiovascular disease, and cancer.
View Dr. Klett’s contributions.
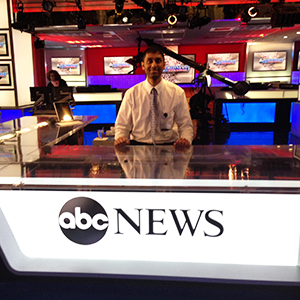
David Clemmons, MD
Dr. David Clemmons’ laboratory focuses on the molecular mechanisms by which the insulin like growth factors (IGF-I and IGF-II) regulate cell proliferation and differentiation, and how these are altered during pathophysiologic processes. His lab has focused on cooperative interactions between multiple signaling networks that regulate growth and differentiation by stimulating a combined activation of multiple signaling pathways whose components are localized on discrete molecular scaffolds at specific intracellular sites. They have determined how the presence of specific physiologic stresses, such as hyperglycemia alter these signaling components to change cellular function in response to these growth factors.
Dr. Clemmons and his lab group have made a conscious effort to extend their basic science findings to translational research applications: they have focused on developing specific monoclonal antibodies that can be used to target molecular interactions that evolve out of cellular stresses. The first of several major discoveries has now progressed to the point where it has been utilized in a phase 2 clinical trial to determine its efficacy in diabetic nephropathy. The team has recruited 127 subjects in this trial which is currently underway and will be completed in 2016. They have developed a second antibody to a separate target that has been shown to be efficacious in animal models of diabetic retinopathy; this antibody is currently being humanized to prepare for a phase 1 clinical trial.
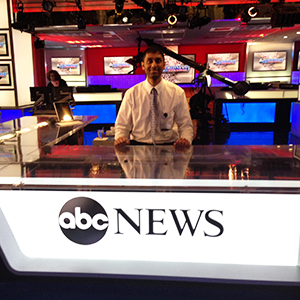
Janet Rubin, MD
Dr. Janet Rubin and her lab group are interested in how the skeleton responds to physical loading. Skeletal mass and architecture are determined largely through the ability of bone cells to respond to daily loading with intelligent remodeling. When mechanical signals are suppressed (no exercise, space travel, getting old) bone structure degenerates leading to fractures. Alternatively, daily skeletal loading (exercise!) leads to bone formation through promoting bone mesenchymal stem cells (“MSCs”) to become osteoblasts. A primary focus of their investigations is to understand the mechanosensory apparatus of the mesenchymal stem cell.
In a sequence of publications the Rubin Lab has shown that mechanical force re-configures the MSC cytoskeleton, repositioning signaling molecules at both the plasma membrane and the nuclear envelope. Signal molecule assembly into focal adhesions then augments mechanical signal generation and intensity. The lab also found that LINC proteins connecting the cytoplasmic cytoskeleton to the inner nucleoskeleton are critical for transmitting mechanical force both between the plasma membrane and the nucleus.
A recent focus in the Rubin laboratory has been to understand how intranuclear actin, which is transported into the nucleus as the cytoskeleton disassembles, can stimulate gene transcription. They surprisingly found that high levels of intranuclear actin strongly promote osteoblastic differentiation of MSCs: this effect caused new bone formation in the bones of mice. This finding might be used to engineer bone in humans (highlighted in a recent Vital Signs).